Research
Research
Our group's aim is to study fundamental physics, by whatever means necessary.
Much of our research takes place as part of the LIGO Laboratory, making more sensitive instruments to better measure gravitational radiation from distance astrophysical sources.
With recent developments in our understanding of quantum measurement, the boundaries of how far we can reach should extend out to the edge of the observable universe and include, in high fidelity, the structure of spacetime at the horizon of black holes.
Intelligent Controls
In terms of complexity, the LIGO interferometers are best imagined as a set of hundreds of feedback loops, which have as sensors and actuators some lasers and mirrors. In order to increase the sensitivity so as to be able to find black holes with thousands of solar masses, and find the pre-merger waves from binary neutron stars, we need to allow the control systems to learn how to fix themselves. But how?
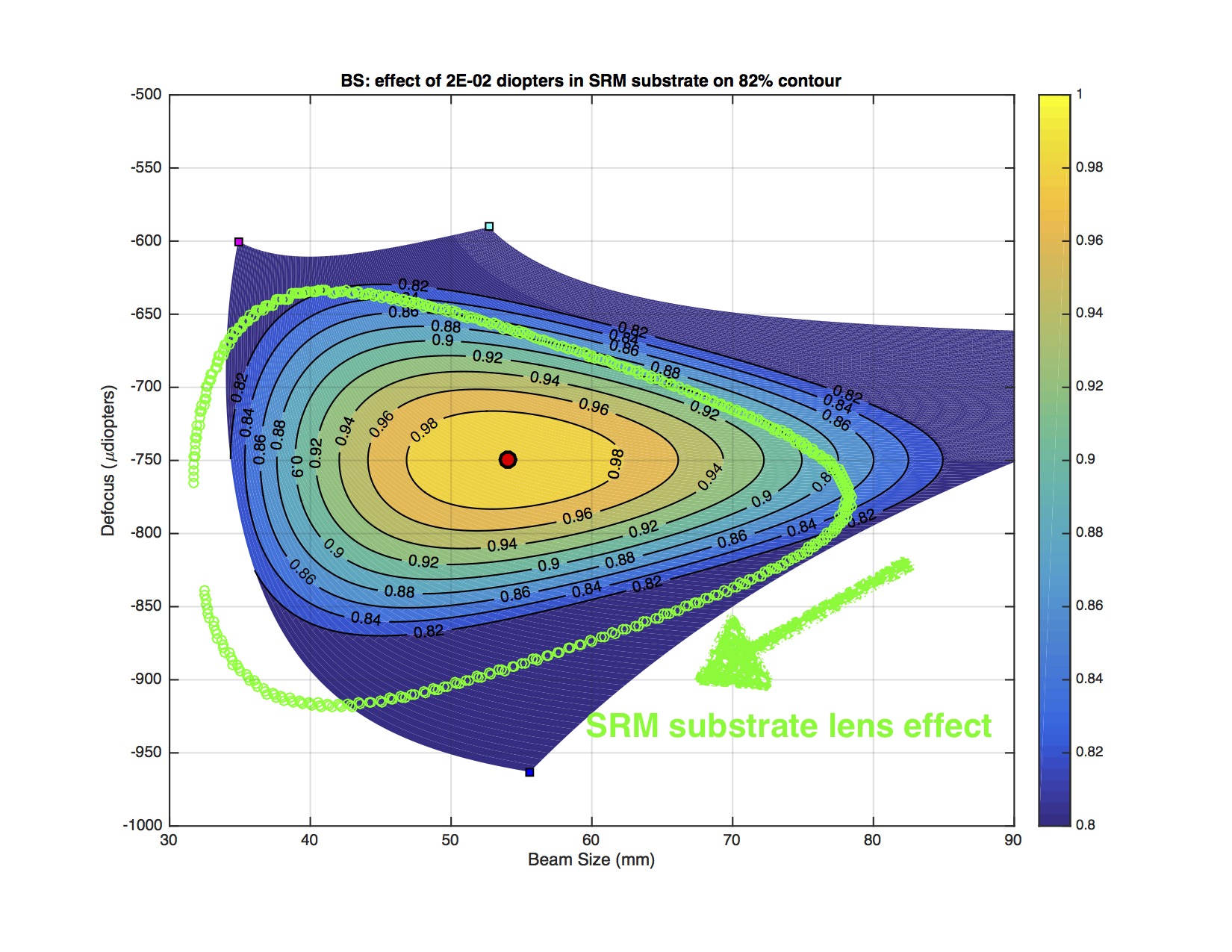
Adaptive Optics
Just as a piano can go out of tune due to changes in temperature and humidity, so too can the optical cavities of the LIGO detectors be poorly matched to each other. In future GW detectors, uncorrected mode-matching losses will severely limit the detector sensitivity due to the effect of decoherence on the quantum entanglement of the laser light. Adaptive optics are required to provide real-time changes to the optical state of the interferometer to compensate for static and dynamic mode-matching errors. They are the piano-tuners of the gravitational-wave world. In collaboration with colleagues at Syracuse and in Australia, we work on designing adaptive optics actuators and sensors, numerical and analytic models of the LIGO cavities and modern state-space controls techniques to operate these systems.
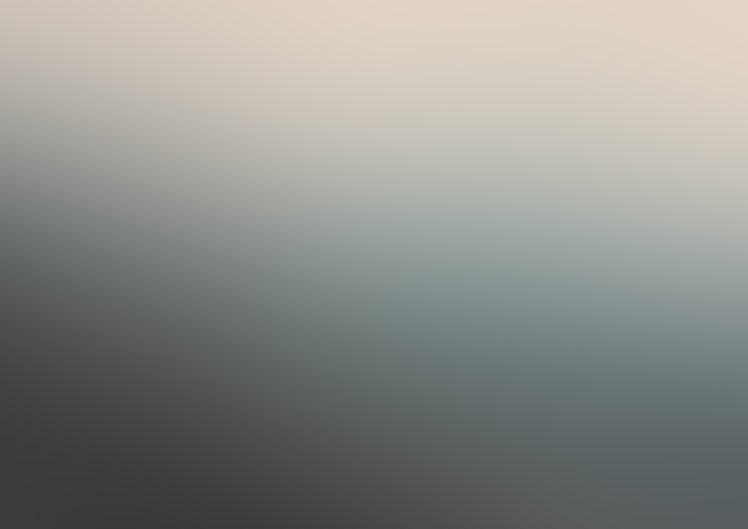
Cryogenic Silicon Interferometers
The best interferometers in the world use glass. In the past several decades the development of silicon has outpaced glass, and now the silicon revolution stands poised to upend the tyranny of glass.
How much more sensitive is an interferometer built out of silicon?
How can we use silicon when we can't see through it?
How does one make a 200 kg silicon mirror?
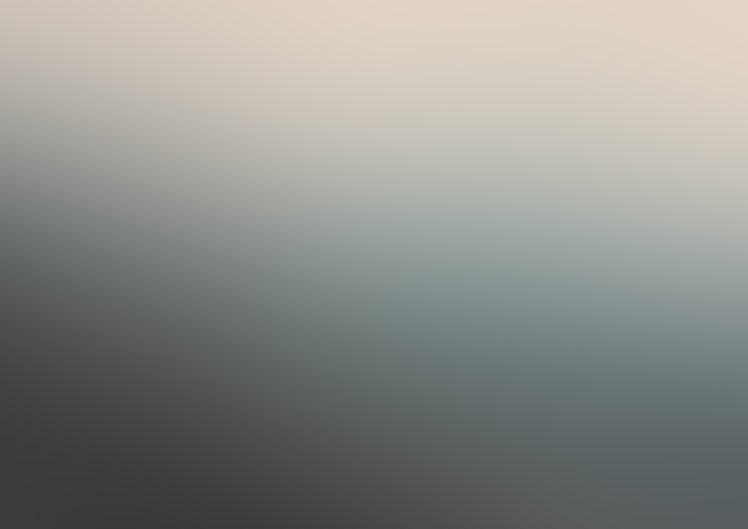
Thermodynamic Limits to Optical Metrology
How precisely can one measure movement and distances?
What are the fundamental thermodynamic limits to optical metrology?
How far below the size of an atom can we see?
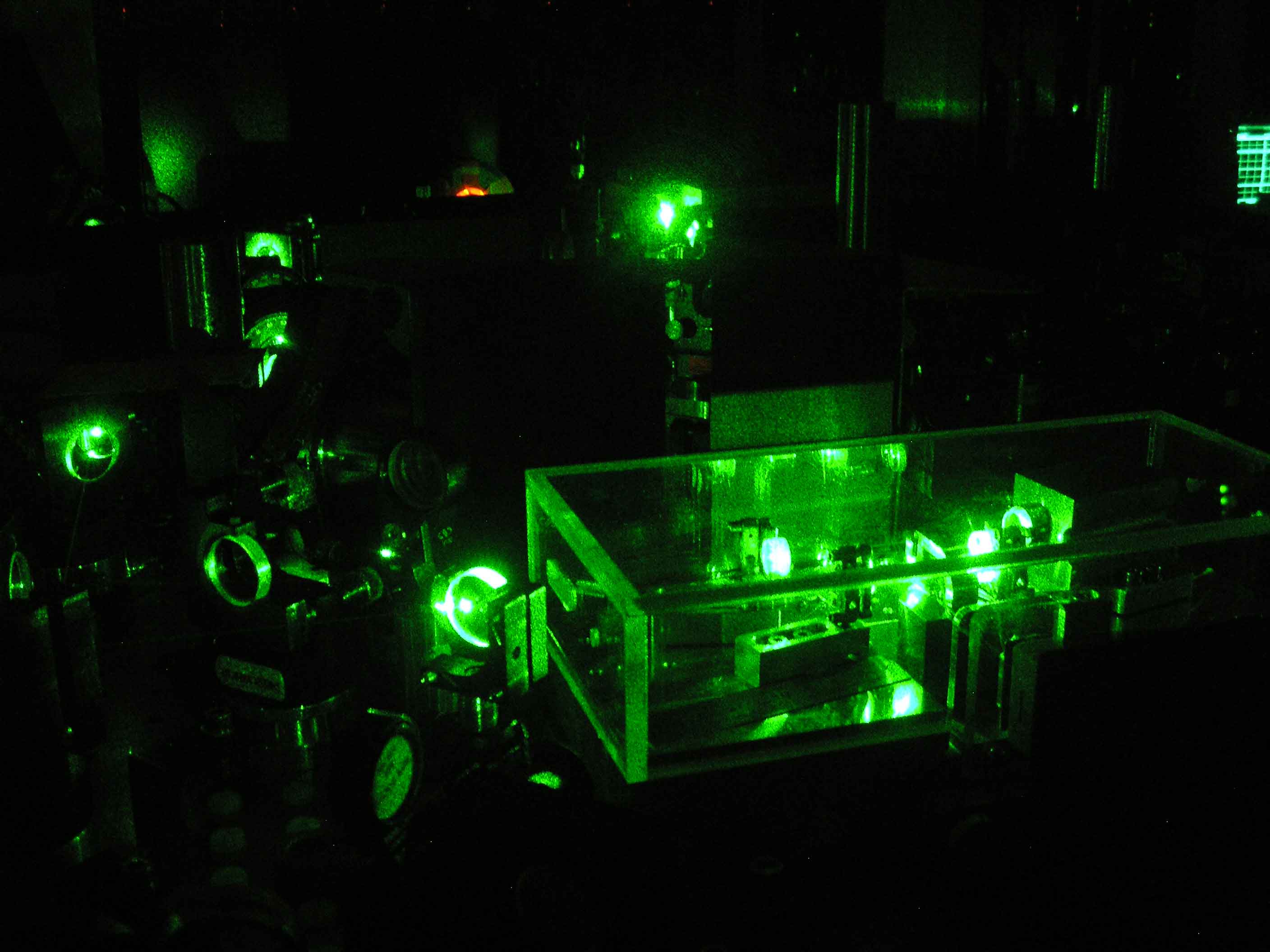
Quantum Information Limits of a Classical Sensor
How precisely is it possible to measure a classical field / force?
Assuming we have a macroscopic quantum sensor, what is the real information extraction bound? For gravitational waves, we can imagine that the signal-to-noise ratio of spacetime strain is only limited by (probably) Planck-scale quantum fuzz. Where do we lose all that SNR? What if we could reach the ultimate quantum bound for conventional sensors (e.g. what's in your phone)?
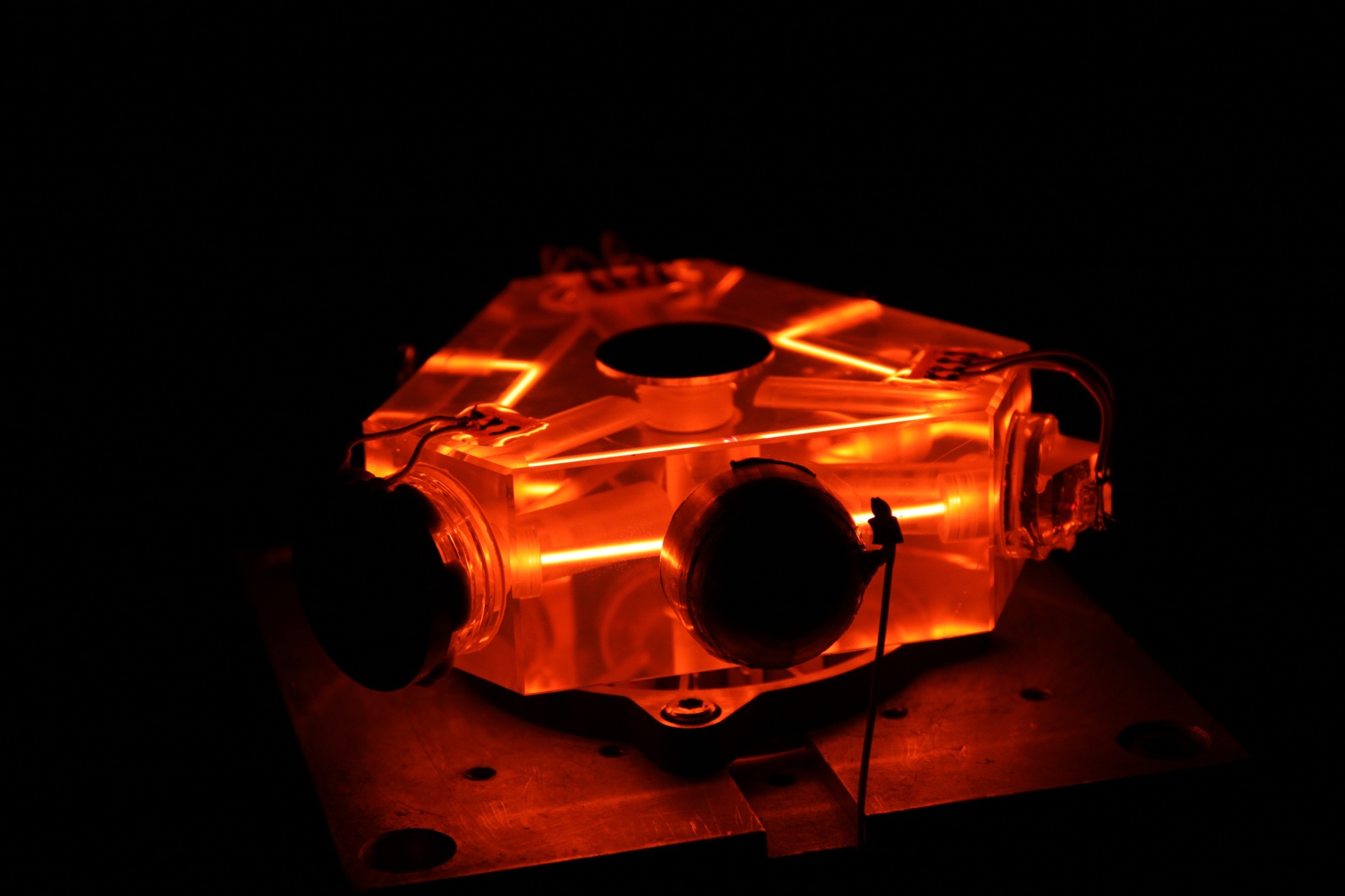
Laser Gyroscope for Rotation Sensing
Laser gyroscopes making use of the Sagnac effect have been used as highly accurate rotation sensors for many years. First used in aerospace and defense applications, these devices have more recently been used for precision seismology and in other research settings. In particular, mid-sized (~1 m-scale) laser gyros have been under development as tilt sensors to augment the adaptive active seismic isolation systems in terrestrial interferometric gravitational wave detectors. The most prevalent design is the 'active' gyroscope, in which the optical ring cavity used to measure the Sagnac degeneracy breaking is itself a laser resonator. We studied a 'passive' approach using a stable cavity illuminated by a stabilized laser source.
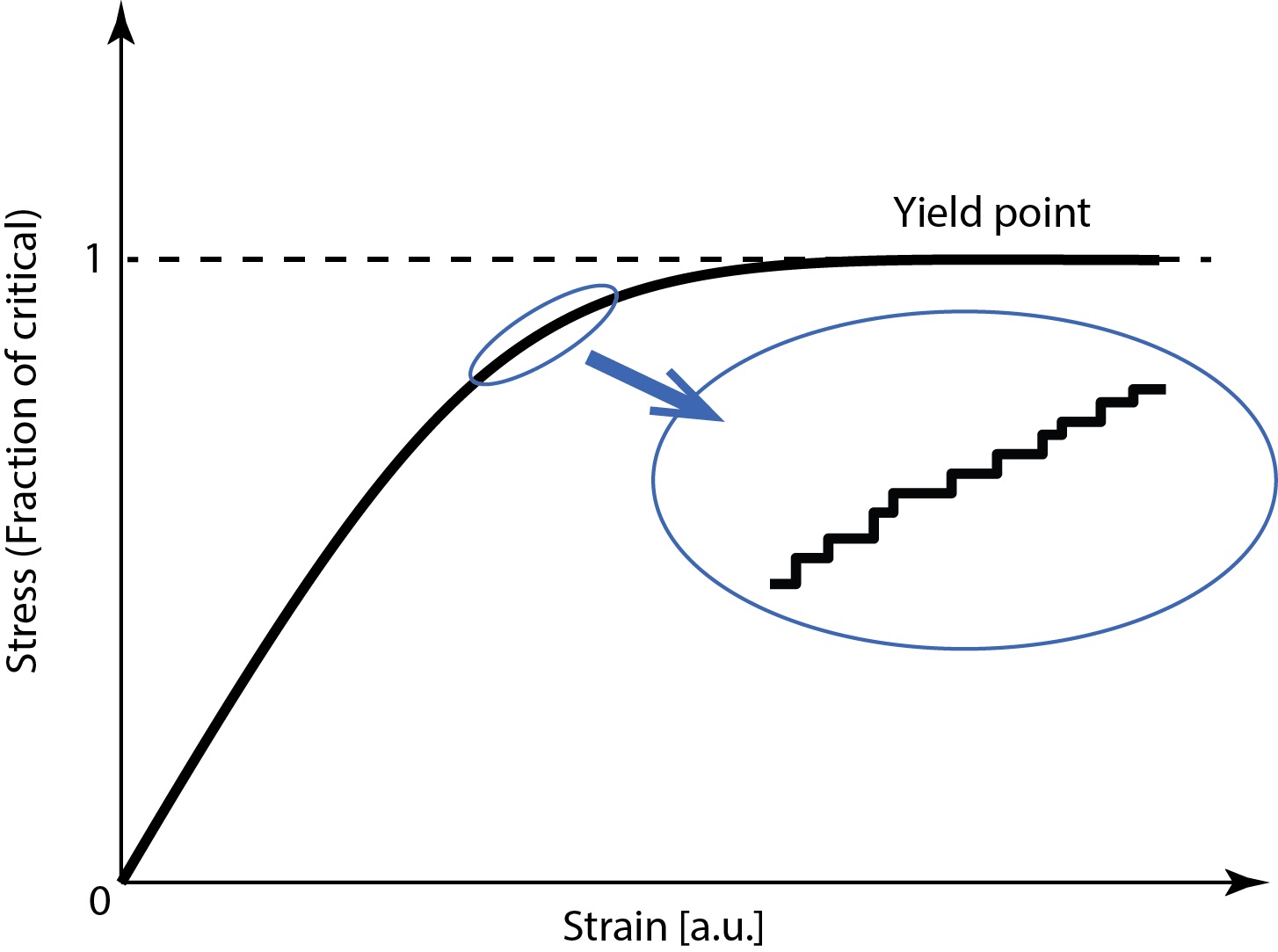
Mechanical Domain Fluctuations
The Advanced LIGO mirror are attached to complex seismic isolation systems, to provide isolation from the ground motion. Some components of the suspension system are cantilever springs made of steel. The goal of the Crackling Noise Experiment is to study the intensity and properties of crackling noise in steel, with a twofold goal: assess the level of noise we expect in Advanced LIGO, and better understand the microscopical foundation of mechanical non-linearities in metals far from the critical yielding point.
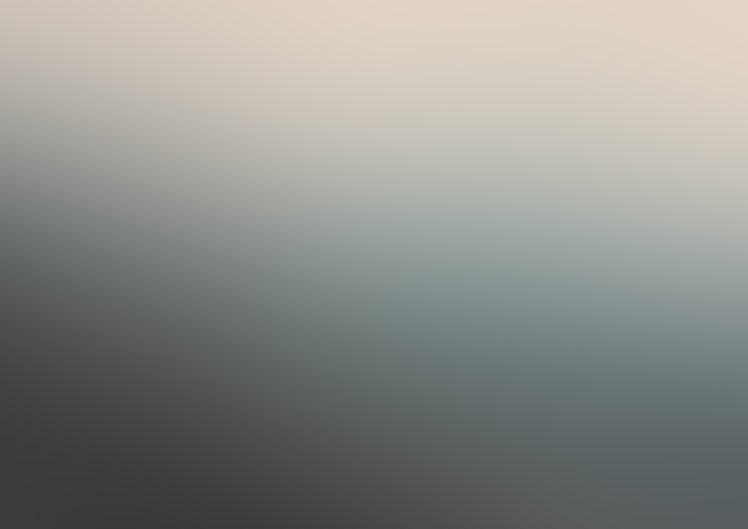
High Q Silicon Opto-Mechanics
Mechanical oscillators with very little internal friction (high Q) are the best way to measure weak classical forces. In order to push the limits in force sensitivity we aim to develop oscillators with Q's of ~ 100 million for eigenfrequencies of hundreds of Hz.
Why?